Adam Hartman
Associate Professor
JWU Faculty Since 2013
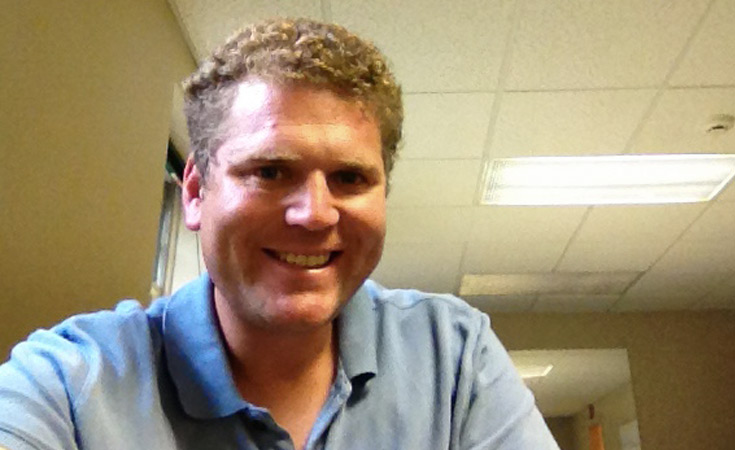
Alan Hartman teaches physics and mathematics in the Science and Math departments at Johnson & Wales University’s Providence Campus.
Hartman has lived in Providence since 2001, when he first moved there as a student in the Ph.D. program in physics at Brown University, which he completed in 2008. Before attending Brown, he was an undergraduate at George Washington University in Washington, D.C., where he triple-majored in physics, mathematics and philosophy.
Hartman has a great number of different research interests. His first real experience working in a lab came during the summer of his sophomore year.
That summer, he joined the research group led by Dr. Mark Reeves at George Washington University, where Hartman carried out experiments that studied the effects of near-field microwaves on thin films. In less technical terms, they looked at how the electrical properties of certain materials (typically semiconducting materials that could be fabricted as very thin films, on the order of a few microns in depth) were affected by the presence of microwave-range electromagnetic radiation. At the time (around 1998), thin film research was an area of great interest, both experimentally and theoretically. This work dovetailed well with another field in physics and engineering that was quickly maturing during this time, and which would become the primary focus of much of Hartman's graduate research in physics: nanotechnology.
When he started graduate school at Brown University in 2001, nanotechnology — the physics and technology of devices fabricated on size-scales of just a few atoms in diameter — was bursting full throttle into the scientific mainstream. Although some of the earliest work in nanotechnology had been done in the early 1990s (for example, the creation of so-called carbon nanotubes), the technology remained somewhat impractical due to a lack of consistency in fabrication methods. But by the time Hartman began his graduate studies, many of the kinks had been worked out, and the fabrication of physical objects (not just carbon nanotubes) had become standardized. This made it possible for higher level experiments to be carried out using various nano-structures.
Hartman's research at Brown began in the purely theoretical realm. Working in the lab of Dr. Jimmy Xu, Hartman developed a theoretical model that explained the nature of electromechanical coupling in single-walled carbon nanotubes. He sought to understand how the bond lengths between the carbon atoms that comprised the wall of the nanotube would respond when a single electron (and then multiple electrons) were injected into the tube and accelerated along its axis. This problem was interesting because single-walled carbon nanotube walls are one carbon atom in thickness, and thus the electrical behavior and mechanical response requires a quantum mechanical description, not a classical one. Consequently, as is often the case in quantum electronics, the resulting phenomena has properties that are considered “exotic” on the macroscopic scale, and which might be exploited to create novel electromechanical devices at the atomic level.
Hartman's initial theoretical work on nanotubes lead into the second part of his graduate research: the fusion of nanotechnology with biophysics. The convergence of biology, physics, computer science, engineering and chemistry has been a prevelant theme over the last two decades. The successful sequencng of the human genome, the standardization of nanofabrication, and the rapid advancements in computational methods have made it possible to begin integrating artificial electronic structures with naturally occuring and “made-to-order” biological molecules. The class of biological molecules that caught Hartman's interest were the so-called “motor proteins.” These proteins (dyenin, kinesin and myosin are a few examples) are molecules that are responsible for, among other things, the rapid active transport of molecules throughout biological systems. They appealed to him as a physicist because their motion and general behvaior was amenable to mathematical modeling by treating them as classical force-generating mechanical engines. Instead of carbon-based fuel, motor proteins use either ATP or GTP as their fuel, and cycle through a number of mechanical steps as they extract energy from this fuel.
Education
- Ph.D., Brown University
- B.S. Physics, George Washington University
- B.S. Math, George Washington University
- B.A. Philosophy, George Washington University
Courses
- PHY1011 General Physics I
- PHY1022 General Physics II
- PHY2011 Physics I
- MATH1020 Algebra
- MATH1040 Calculus I
- ILS2280 Science and Civilization